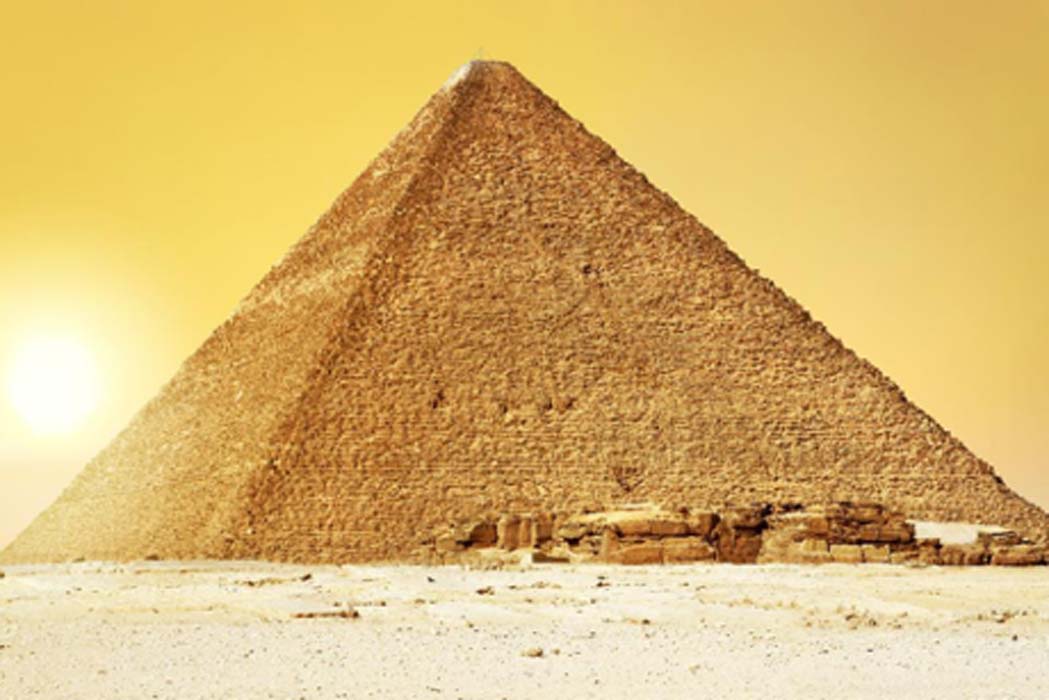
Discovery of a big void in Khufu’s Pyramid by observation of cosmic-ray muons, updated with interactive links to data sets at the bottom
Abstract
The Great Pyramid, or Khufu’s Pyramid, was built on the Giza plateau in Egypt during the fourth dynasty by the pharaoh Khufu (Cheops)1, who reigned from 2509 BC to 2483 BC. Despite being one of the oldest and largest monuments on Earth, there is no consensus about how it was built2,3. To understand its internal structure better, we imaged the pyramid using muons, which are by-products of cosmic rays that are only partially absorbed by stone4,5,6. The resulting cosmic-ray muon radiography allows us to visualize the known and any unknown voids in the pyramid in a non-invasive way. Here we report the discovery of a large void (with a cross-section similar to that of the Grand Gallery and a minimum length of 30 metres) situated above the Grand Gallery. This constitutes the first major inner structure found in the Great Pyramid since the nineteenth century1. The void, named ScanPyramids’ Big Void, was first observed with nuclear emulsion films7,8,9 installed in the Queen’s chamber, then confirmed with scintillator hodoscopes10,11 set up in the same chamber and finally re-confirmed with gas detectors12 outside the pyramid. This large void has therefore been detected with high confidence by three different muon detection technologies and three independent analyses. These results constitute a breakthrough for the understanding of the internal structure of Khufu’s Pyramid. Although there is currently no information about the intended purpose of this void, these findings show how modern particle physics can shed new light on the world’s archaeological heritage.
Access options
Subscribe to Journal
Get full journal access for 1 year
£198.00
only £3.81 per issue
All prices include VAT for United Kingdom.
Rent or Buy article
Get time limited or full article access on ReadCube.
from$8.99
All prices are NET prices.
Additional access options:
References
1
Lehner, M. The Complete Pyramids: Solving the Ancient Mysteries (Thames and Hudson, 2008)
2
Hawass, Z. Pyramid construction: new evidence discovered in Giza. In Stationen. Beiträge zur Kulturgeschichte Ägyptens Rainer Stadelmann gewidmet (eds Guksch, H. & Polz, D. ) 53–62 (Philipp von Zabern, 1998)
3
Smith, C. B ., Hawass, Z. & Lehner, M. How the Great Pyramid was Built (Harper Collins, 2006)
4
Particle Data Group. Review of particle physics. Chin. Phys. C40, 100001 (2016)
5
Alvarez, L. W. et al. Search for hidden chambers in the pyramids. Science 167, 832–839 (1970)
6
Tanaka, H. K. M., Nakano, T., Takahashi, S., Yoshida, J. & Niwa, K. Development of an emulsion imaging system for cosmic-ray muon radiography to explore the internal structure of a volcano, Mt. Asama. Nucl. Instrum. Methods A 575, 489–497 (2007)
7
Morishima, K., Nishio, A., Moto, M., Nakano, T. & Nakamura, M., Development of nuclear emulsion for muography. Ann. Geophys. 60, 0112 (2017)
8
Nakamura, T. A. et al. The OPERA film: new nuclear emulsion for large-scale, high-precision experiments. Nucl. Instrum. Methods A 556, 80–86 (2006)
9
Nishio, A., Morishima, K., Kuwabara, K. & Nakamura, M. Development of nuclear emulsion detector for muon radiography. Phys. Proc. 80, 74–77 (2015)
10
Fujii, H. et al. Detection of on-surface objects with an underground radiography detector system using cosmic-ray muons. Progr. Theor. Exp. Phys. 5, 053C01 (2017)
11
Fujii, H. et al. Performance of a remotely located muon radiography system to identify the inner structure of a nuclear plant. Progr. Theor. Exp. Phys. 7, 073C01 (2013)
12
Bouteille, S. et al. A Micromegas-based telescope for muon tomography: the WatTo experiment. Nucl. Instrum. Methods A 834, 223–228 (2016)
13
Dash, G. The Great Pyramid’s footprint: results from our 2015 survey. Aeragram 16, 8–14 (2015)
14
Hawass, Z. et al. First report: video survey of the southern shaft of the Queen’s chamber in the Great Pyramid. Ann. Serv. Antiq. Egypte 84, 203–216 (2010)
15
Richardson, R. et al. The “Djedi” robot exploration of the southern shaft of the Queen’s Chamber in the Great Pyramid of Giza, Egypt. J. Field Robot. 30, 323–348 (2013)
16
Tallet, P. Les Papyrus de la Mer Rouge 1 : Le “Journal de Merer” http://www.ifao.egnet.net/publications/catalogue/9782724707069/ (Institut Français d’Archéologie Orientale, 2017)
17
Bui, H. D. Imaging the Cheops Pyramid (Springer Science & Business Media, 2011)
18
Butler, D. K. Microgravimetric and gravity gradient techniques for detection of subsurface cavities. Geophysics 49, 1084–1096 (1984)
19
Yoshimura, S ., Nakagawa, T ., Tonouchi, S. & Seki, K. Non-destructive pyramid investigation. Parts 1 and 2. Stud. Egypt. Cult. 6 & 8, http://www.egyptpro.sci.waseda.ac.jp/pdf%20files/SEC06%20Pyramid%20Investigation1.pdf and http://www.egyptpro.sci.waseda.ac.jp/pdf%20files/SEC08%20Pyramid%20Investigation2.pdf (1987)
20
Morishima, K . et al. First demonstration of cosmic ray muon radiography of reactor cores with nuclear emulsion based on an automated high-speed scanning technology. Proc. 26th Worksh. on ‘Radiation Detectors and Their Uses’ 27–36, https://lib-extopc.kek.jp/preprints/PDF/2012/1225/1225008.pdf (2012)
21
Borozdin, K. N. et al. Surveillance: radiographic imaging with cosmic-ray muons. Nature 422, 277 (2003)
22
Menichelli, M. et al. A scintillating fibres tracker detector for archaeological applications. Nucl. Instrum. Methods A 572, 262–265 (2007)
23
Saracino, G. et al. Imaging of underground cavities with cosmic-ray muons from observations at Mt. Echia (Naples). Sci. Rep. 7, 1181 (2017)
24
Menchaca-Rocha, A. Searching for cavities in the Teotihuacan Pyramid of the Sun using cosmic muons experiments and instrumentation. Int. Cosmic Ray Conf. 4, 325 (2011)
25
Yoshimoto, M., Nakano, T., Komatani, R. & Kawahara, H. Hyper-track selector nuclear emulsion readout system aimed at scanning an area of one thousand square meters. Progr. Theor. Exp. Phys. 10, 103H01 (2017)
26
Morishima, K., Hamada, K., Komatani, R., Nakano, T. & Kodama, K. Development of an automated nuclear emulsion analyzing system. Radiat. Meas. 50, 237–240 (2013)
27
Hamada, K. et al. Comprehensive track reconstruction tool “NETSCAN 2.0” for the analysis of the OPERA Emulsion Cloud Chamber. J. Instrum. 7, P07001 (2012)
28
Agostinelli, S. et al. geant4—a simulation toolkit. Nucl. Instrum. Methods A 506, 250–303 (2003)
29
Morishima, K. Latest developments in nuclear emulsion technology. Phys. Proc. 80, 19–24 (2015)
30
Nishio, A . et al. Long term property of nuclear emulsion. Progr. Proc. 1st Int. Conf. on ‘Advanced Imaging’ (ICAI2015) 668–671 (Imaging Society of Japan, 2015)
31
Aoki, S. et al. The fully automated emulsion analysis system. Nucl. Instrum. Methods B 51, 466–472 (1990)
32
Morishima, K. & Nakano, T. Development of a new automatic nuclear emulsion scanning system, S-UTS, with continuous 3D tomographic image read-out. J. Instrum. 5, P04011 (2010)
33
Miyake, S. Rapporteur paper on muons and neutrinos. 13th Int. ‘Cosmic Ray’ Conf. Vol. 5, 3638–3655 (1973)
34
Dormion, G. La Chambre de Cheops: Analyse Architecturale (Fayard, 2004)
35
Reyna, D. A simple parameterization of the cosmic-ray muon momentum spectra at the surface as a function of zenith angle. Preprint at https://arxiv.org/abs/hep-ph/0604145 (2006)
36
Jokisch, H., Carstensen, K., Dau, W., Meyer, H. & Allkofer, O. Cosmic-ray muon spectrum up to 1 TeV at 75 zenith angle. Phys. Rev. D 19, 1368 (1979)
37
Giomataris, I. Y., Rebourgeard, P., Robert, J. P. & Charpak, G. MICROMEGAS: a high-granularity position-sensitive gaseous detector for high particle-flux environments. Nucl. Instrum. Methods A 376, 29–35 (1996)
38
Giomataris, I. Y. et al. Micromegas in a bulk. Nucl. Instrum. Methods A 560, 405–408 (2006)
39
Alexopoulos, T. et al. A spark-resistant bulk-micromegas chamber for high-rate applications. Nucl. Instrum. Methods A 640, 110–118 (2011)
40
Procureur, S ., Dupré, R. & Aune, S. Genetic multiplexing and first results with a 50 × 50 cm2 Micromegas. Nucl. Instrum. Methods A 729, 888–894 (2013)
41
Flouzat, C . et al. Dream: a 64-channel front-end chip with analogue trigger latency buffer for the Micromégas tracker of the CLAS12 experiment. Proc. TWEPP Conf. https://indico.cern.ch/event/299180/contributions/1659618/contribution.pdf (2014)
42
Brun, R. & Rademakers, F. ROOT—an object oriented data analysis framework. Nucl. Instrum. Methods A 389, 81–86 (1997)
43
Maragioglio, V. & Rinaldi, C. L’Architettura delle Piramidi Menfite. Parte IV, La Grande Piramide di Cheope (Rapallo, 1965)
44
Kraus, K. Photogrammetry: Geometry from Images and Laser Scans (Walter de Gruyter, 2007)
Acknowledgements
This experiment is part of the ScanPyramids project, which is supported by NHK, La Fondation Dassault Systèmes, Suez, IceWatch, le Groupe Dassault, Batscop, Itekube, Parrot, ILP, Kurtzdev, Gen-G and Schneider Electric. Measurement with nuclear emulsions was supported by the JST-SENTAN Program from the Japan Science and Technology Agency and JSPS KAKENHI (grant JP15H04241). The CEA telescopes were funded partly by the Région Ile-de-France and the P2IO LabEx (grant ANR-10-LABX-0038) in the framework ‘Investissements d’Avenir’ (grant ANR-11-IDEX-0003-01) managed by the Agence Nationale de la Recherche (France). The detectors were built by the ELVIA company and the CERN Micro-Pattern Gaseous Detector workshop. We thank the members and benefactors of the ScanPyramids project, and in particular: T. Hisaizumi, the members of Cairo University, the members of the F-laboratory in Nagoya University, Y. Doki, the Aïn El Shams University 3D scanning team, the members of the Egyptian Ministry of Antiquities, K. El Enany, M. El Damaty, T. Tawfik, S. Mourad, S. Tageldin, E. Badawy, M. Moussa, T. Yabuki, D. Takama, T. Shibasaki, K. Tsutsumida, K. Mikami, J. Nakao, H. Kurihara, S. Wada, H. Anwar, T. de Tersant, P. Forestier, L. Barthès, M.-P. Aulas, P. Daloz, S. Moignet, V. Raoult-Desprez, S. Sellam, P. Johnson, J.-M. Boursier, T. Alexandre, V. Ferret, T. Collet, H. Andorre, C. Oger-Chevalier, V. Picou, B. Duplat, K. Guilbert, J. Ulrich, D. Ulrich, C. Thouvenin, L. Jamet, A. Kiner, M.-H. Habert, B. Habert, L. Gaudé, F. Schuiten, F. Barati, P. Bourseiller, R. Theet, J.-P. Lutgen, R. Chok, N. Duteil, F. Tran, J.-P. Houdin, L. Kaltenbach, M. Léveillé-Nizerolle, R. Breitner, R. Fontaine, H. Pomeranc, F. Ruffier, G. Bourge, R. Pantanacce, M. Jany, L. Walker, L. Chapus, E. Galal, H. A. Mohalhal, S. M. Elhindawi, J. Lefaucheux, J.-M. Conan, E. M. Elwilly, A. Y. Saad, H. Barrada, E. Priou, S.Parrault, J.-C. Barré, X. Maldague, C. Ibarra Castenado, M. Klein, F. Khodayar, G. Amsellem, M. Sassen, C. Béhar, M. Ezzeldin, E. Van Laere, D. Leglu, B. Biard, N. Godin, P. der Manuelian, L. Gabriel, P. Attar, A. De Sousa, F. Morfoisse, R. Cotentin, C. Delache and G. Perrin.
Author information
Affiliations
F-lab, Nagoya University, Furo-cho, Chikusa-ku, Nagoya, 464-8602, Aichi, Japan
Kunihiro Morishima
, Mitsuaki Kuno
, Akira Nishio
, Nobuko Kitagawa
, Yuta Manabe
& Masaki Moto
High Energy Accelerator Research Organization (KEK), 1-1 oho, Tsukuba, Ibaraki, 305-0801, Japan
Fumihiko Takasaki
, Hirofumi Fujii
, Kotaro Satoh
, Hideyo Kodama
, Kohei Hayashi
& Shigeru Odaka
Institut de Recherche sur les lois Fondamentales de l’Univers (IRFU), Commissariat à l’Energie Atomique et aux Energies Alternatives (CEA), Université Paris Saclay, Gif-sur-Yvette, 91191, France
Sébastien Procureur
, David Attié
, Simon Bouteille
, Denis Calvet
, Christopher Filosa
, Patrick Magnier
, Irakli Mandjavidze
& Marc Riallot
HIP Institute, 50 rue de Rome, Paris, 75008, France
Benoit Marini
, Vincent Steiger
, Nicolas Serikoff
, Hany Helal
& Mehdi Tayoubi
Emissive, 71 rue de Provence, Paris, 75009, France
Pierre Gable
& Emmanuel Guerriero
NHK Enterprises, Inc. (NEP), 4-14 Kamiyama-cho, Shibuya-ku, Tokyo, 150-0047, Japan
Yoshikatsu Date
Suave Images, N-2 Maison de Shino, 3-30-8 Kamineguro, Meguro-Ku, 153-0051, Tokyo, Japan
Makiko Sugiura
Cairo University, 9 Al Gameya, Oula, Giza Governorate, Egypt
Yasser Elshayeb
, Mustapha Ezzy
& Hany Helal
Ain Shams University, Kasr el-Zaafaran, Abbasiya, Cairo, Egypt
Tamer Elnady
Inria, Villers-lès-Nancy, F-54600, France
Jean-Baptiste Mouret
CNRS, Vandœuvre-lès-Nancy, F-54500, France
Jean-Baptiste Mouret
Université de Lorraine, Vandœuvre-lès-Nancy, F-54500, France
Jean-Baptiste Mouret
Dassault Systèmes, 10 Rue Marcel Dassault, Vélizy-Villacoublay, 78140, France
Bernard Charlès
& Mehdi Tayoubi
Contributions
K.M., M.K., A.N., N.K., Y.M. and M.M. performed the experiments and analysed the results for the nuclear emulsion films; F.T., H.F., K.S., H.K., K.H. and S.O. performed the experiments and analysed the results for the scintillator hodoscopes. S.P., D.A., S.B., D.C., C.F., P.M., I.M. and M.R. performed the experiment and analysed the results from the gas detector telescopes. B.M., P.G., E.G., N.S., Y.D. and M.S. created the 3D models used for the muography simulations and the RTMS. B.M. designed and implemented the RTMS and contributed to the analyses. Y.E., T.E., M.E. and V.S. coordinated the different experimental operations in the field (muography, 3D scans).The paper was mainly written by K.M., S.P., F.T., M.T., B.M. and J.-B.M., with contributions from all the other authors. H.H., M.T., B.C., B.M. and Y.E. designed and coordinated the project (ScanPyramids).
Corresponding authors
Correspondence to Kunihiro Morishima or Mehdi Tayoubi.
Ethics declarations
Competing interests
The authors declare no competing financial interests.
Additional information
Reviewer Information Nature thanks G. Saracino, L. Thompson and the other anonymous reviewer(s) for their contribution to the peer review of this work.
Publisher's note: Springer Nature remains neutral with regard to jurisdictional claims in published maps and institutional affiliations.
Extended data figures and tables
a, A cross-sectional schematic view of a nuclear emulsion. b, Enlarged schematic view of the emulsion layer. Silver bromide crystals are dispersed in gelatin. The red dashed arrow shows the trajectory of the charged particle. c, After the photographic development process, silver grains are aligned along the trajectory (track) of the charged particle. d, An optical microscopic photograph of the track of a minimum ionizing particle recorded in a nuclear emulsion. e, A nuclear emulsion after development. f, A vacuum-packed nuclear emulsion. g, Schematic view of the detector configuration: six packed nuclear emulsion films with a detection area of 30 cm × 25 cm each (yellow) are fixed between aluminium supporting plates (honeycomb plate, in grey). Two films stacked on top of each other are pressed with a rubber sheet (black) by four short screws. Three additional long screws are used as legs to correct the inclination of the detector. h, Cross-sectional schematic view of the nuclear emulsion detector as shown in g. Two packed films are stacked between two honeycomb plates and rubber sheet.
Each panel shows slices for tanθy every 0.25 units (in tangent) and separated into four ranges at 0 ≤ tanθy < 1 (see Fig. 2a–d). The top part of each panel shows muon flux distribution and the bottom part of each panel shows the difference of muon flux. In the top part of each panel, the red line shows the data, the black solid line shows the simulation with the internal structures, and the grey dashed line shows the simulation without any internal structure. In the bottom part of each panel, the red line shows the subtraction between the data and the simulation with the internal structures, and the black line shows subtraction between the simulation with and without the internal structures, so that the Grand Gallery appears as a muon excess. Error bars indicate statistical error of 1σ (standard deviation). The comparison between the excess that corresponds to the Grand Gallery and the one that corresponds to the new void shows that the two structures are of a similar scale. For each projection of difference of muon flux, we performed a Gaussian fitting to estimate the direction of anomalies. The fitting zone was 0 ≤ tanθx ≤ 0.2 for position NE1 and −0.2 ≤ tanθx ≤ 0 for position NE2. These fitted centres were used for the triangulation.
a, Vertical view of the detector, consisting of two units of orthogonal double scintillator layers. A blue arrow indicates a muon track passing through the whole instrument. b, Cross-section of a scintillator element, showing the central hole for the optical fibre. c, Grid made of double layers, detecting the position of incident muons. d, Plane view of the detector, with an active area of 1.2 m × 1.2 m.
Left, relative yield of the measurement to the simulation (including known structures) at position H1 for four slices (the width of each bin is 24 Δy). Right, relative yield at position H2 for five slices (the width of each bin is 16 Δy). Error bars show statistical errors of 1σ (standard deviation).
a, Design of a telescope (without its cover) showing the four detectors, the electronics box, the battery and the gas bottles. b, Design of the multiplexed Micromegas detector. c, Principle of a Micromegas detector, showing the ionization and amplification of the signal initiated from a charged particle (dotted array). d, Layout of the detector with the micromesh in red, the resistive strip film in blue, and the Y and X copper readout strips in yellow. HV, high voltage; ADC, analogue digital converter. e–g, Amplitude variation of a detector in the Alhazen telescope as a function of time for two previous campaigns (ScanPyramids missions 1 and 2) and the one reported here (g), showing the effect of the patented feedback. Large variations (as observed in e and f) can lead to inefficiency or degraded resolution, and are totally absent from the data of this paper. The only step observed in g corresponds to a manual change of the target amplitude. h, Typical signal recorded in a detector, where each line corresponds to an electronic channel.
The slices are 0.10 (in tangent) thick, and each slice is shifted by 0.02 with respect to the previous one, which means they overlap. Distributions are generally smooth, with two large muon excesses on histograms 5, 6 and 15 (see Methods). Error bars show statistical error of 1σ (standard deviation).
a, RTMS output, showing the CEA position G2 (Alhazen) sensor with a 6-view layout. Interactive view with display of sensor field of view and cone projections. Real-time simulation with internal structure overlaid in wireframe. Result with sensor point-of-view superposition. b, Zoom on chevron area (shaded wireframe). c, Enlarged view of optimized 3D model (shaded wireframe). d, Detail of optimized 3D model.
Extended Data Table 1 Comparison of the three muon detection technologies
PowerPoint slides